ARCHAEOLOGICAL MATERIALS
Most archaeological materials are susceptible to chemical and physical change during burial. The type and extent of this change, usually referred to as decay, will depend on many factors relating to the finds and the burial environment. A knowledge of archaeological materials and their likely interactions with the environment can help to predict their survival on a site.
Understanding decay in the burial environment can also explain the altered appearance of finds and materials as compared to their more recognisable form pre-burial. This is important for the identification of finds on site which allows the correct first aid for finds actions to be taken.
Types of Archaeological Material
Broad categorisation of archaeological materials is based on their chemical composition and will be either inorganic, metallic or organic. Metals are also inorganic materials but their distinct structures and chemical bonding, which define their material properties, mean it is helpful to consider them as a separate category.
Descriptions and examples of these material types are given in Table 2.1.
Table 2.1 Descriptions and examples of inorganic, metal and organic archaeological materials
A find may be made of a single material or be a composite of different materials (Figure 2.1). Examples of composite finds and the materials they comprise are given in (Table 2.2):
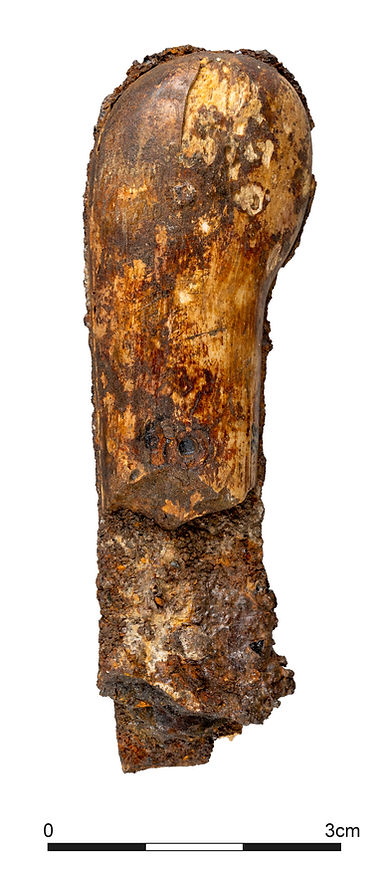
Figure 2.1 An iron knife with a bone handle showing corrosion of the iron and staining of the bone (Image: Cardiff University)
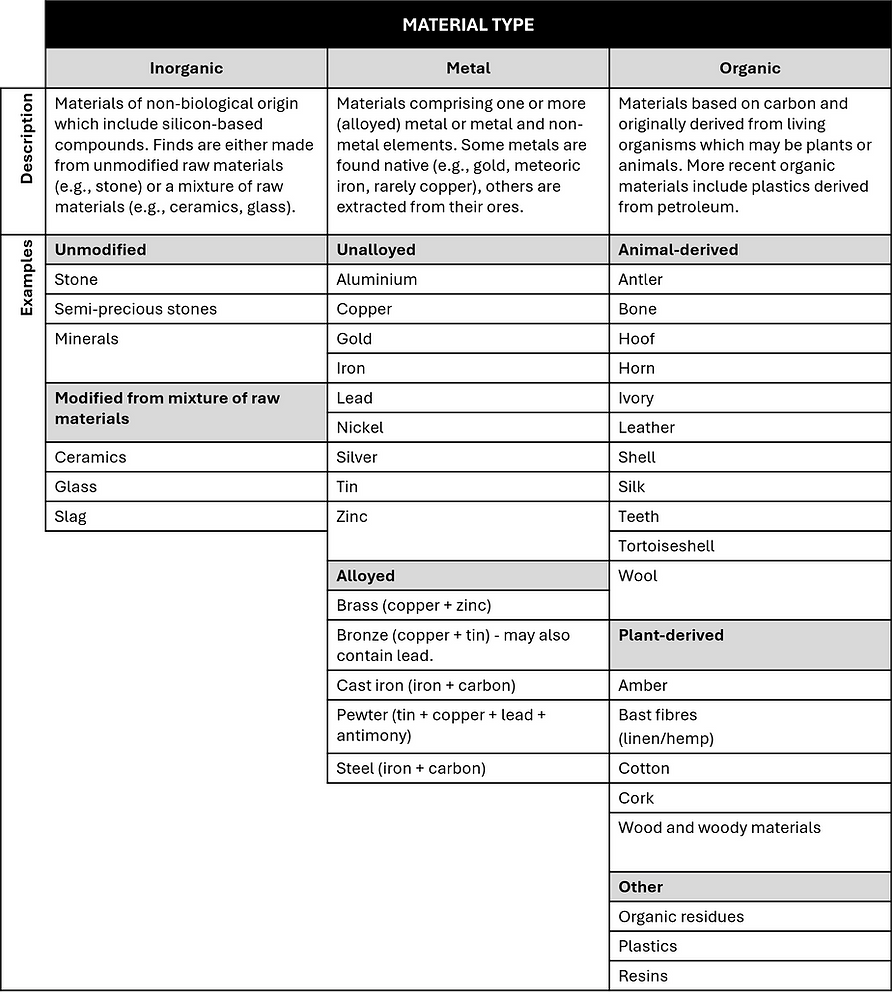
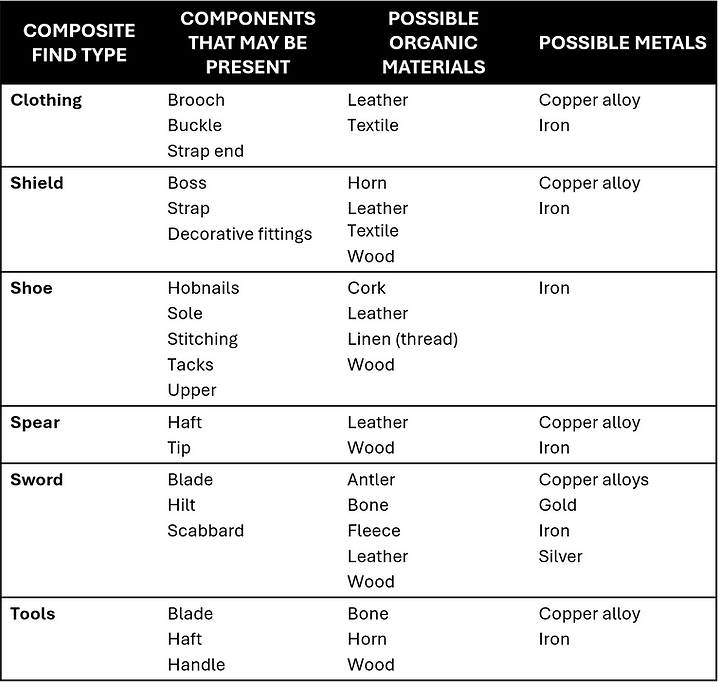
Table 2.2 Examples of composite finds and their materials
Decay of Materials & Finds During Burial
Although materials may be familiar in an undecayed state, very rarely will a find be excavated in an unaltered condition. These changes can create difficulties in identifying materials and finds on excavation (Figure 2.2). The extent and nature of change that occurs to finds during burial will depend on:
-
Intrinsic object factors
-
Material(s)
-
Manufacture
-
Condition on burial
-
-
Burial environment parameters
-
Water content and movement
-
Oxygen levels
-
pH (acidity/alkalinity)
-
Temperature
-
Soluble salts
-
Microorganism activity
-
Physical disturbance
-
-
Presence of other materials
Influence of intrinsic object factors on decay
Material
Examining the broad material categories can allow generalised statements about their decay to be made. These can act as a guide, but the decay of each find will be influenced by the many factors explored here.
Manufacture
Decay routes will be specific to materials and burial environments but differences between the manufacturing processes of similar materials can have implications for decay. Examples of this include ceramics and glass.
Ceramics (Figure 2.3): The composition of the raw materials (clay minerals and additives) of a ceramic are influential but the most important factor affecting its decay or survival in the burial environment is likely to be the temperature at which it was fired. High-fired ceramics have a fused structure which is chemically resistant and has minimal pore spaces for ingress of water. Low-fired ceramics have an open structure into which water can ingress, resulting in physical damage (such as from freeze-thaw action). Very low-fired ceramics may be plasticised by water, reverting to a pliable structure which can become deformed by pressure during burial.

Figure 2.2 An example of decay creating difficulties for identification in the case of corroded pewter (Image: Cardiff University)
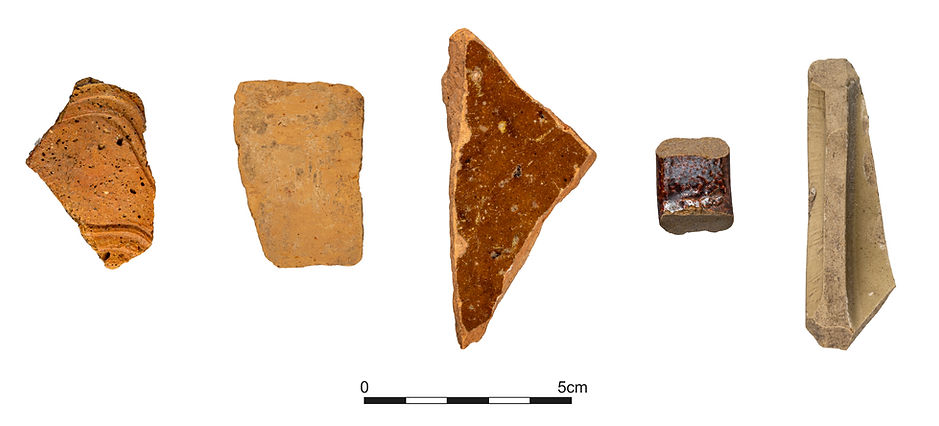
Figure 2.3 Examples of different archaeological ceramics (left-right): incised coarse earthenware; low-fired, well-worn earthenware; lead glazed earthenware; glazed stoneware; salt glazed stoneware (Image: Cardiff University)
Glass (Figure 2.4): Decay of glass is linked to its composition based on the raw materials of its production (silica from sand or stone with fluxes from plant ashes or minerals). Medieval glass (~9-16th centuries) is particularly susceptible to decay due to the use of a potash flux (containing potassium) instead of the soda ash flux (containing sodium) which was common during the Roman period and high lime (calcium-rich) post-medieval glass. Potassium is leached more easily from the silica matrix, leading to increased deterioration of medieval glass.

Condition on burial
Where decay of a material has occurred before burial, this can lead to accelerated decay in the burial environment. For example, wood which has been decayed by fungi prior to burial has a more open structure which allows access for microorganisms in the burial environment. This increases the rate of decay of the wood. Ceramics which have been used to store salts during their use-life are likely to contain soluble salts in their structure. With changes in the availability of water in the burial environment and post-excavation, this can lead to physical damage by volume changes in the salts.
Influence of burial environment parameters on decay
Burial environment parameters are dictated by a multitude of factors, including underlying geology, topography, local vegetation, land use, and climate. These factors will determine the nature and extent of the agents of decay acting on archaeological materials.
Water
Water is a major agent of decay for many archaeological materials due to its polarity and ability to dissolve compounds (such as soluble salts). In aerated soils, it supports corrosion of metals and biological deterioration of organic materials. In waterlogged environments, it can support the survival of organic materials by limiting the availability of oxygen required for most biological processes. Movement of water through a soil may physically erode buried materials, including inorganics.
Soils are classified by division into categories according to their particle size (Table 2.3). The smaller the particle size in a soil, the closer the packing of the particles and the smaller the pores between them. The smaller the pore size, the greater the ability of the soil to hold water due to capillarity. This means that gravels and coarse sands tend to be more free-draining and have lower water content than finer sands and silts, depending on the position of the water table.
Figure 2.4 Archaeological glass showing the alteration of its surface with characteristic irridescence (Image: Cardiff University)
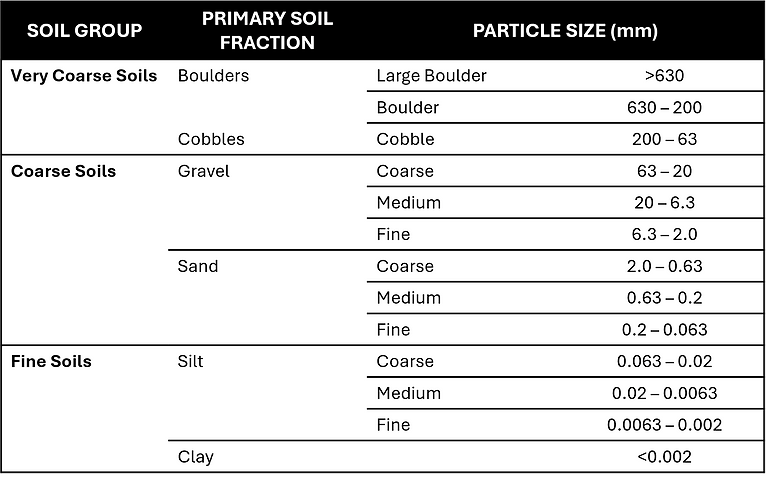
Table 2.3 Description of soils (after British Standard BS 5930:2015+A1:2020)
Oxygen
Oxygen will be present in the burial environment either in air-filled spaces between soil particles, or in lower quantities dissolved in soil water. Where soil particle sizes are larger and water drains more freely, oxygen will be more plentiful. Oxygen is essential for many decay reactions in the burial environment, including corrosion reactions of metals and most biodeterioration reactions of organic materials.
pH
A measure of hydrogen ions in solution, pH indicates acidity or alkalinity. The pH range of UK soils is between 3 (acid) and 9 (weakly alkaline) depending on geology, vegetation and human activity, with most being mildly to moderately acidic. Acidic or alkaline conditions may either preserve or cause decay of a material during burial depending on its chemistry (Table 2.4).
Temperature
The temperature of a soil is variable with depth, fluctuating less on a daily and seasonal basis with increasing soil depth. This means that shallower deposits will experience a greater range of temperature change. Temperature is linked to rates of chemical reactions occurring in the burial environment with reactions proceeding more quickly as temperature increases. Cold environments limit decay reactions but occasional low temperatures in damp burial contexts may contribute to physical damage through freeze-thaw action.
Soluble Salts
Soluble salts are inherent in some porous inorganic materials (such as limestone), may be introduced during use-life of an object (such as ceramic salt containers) or may derive from the burial environment (arising from local geology or human activity). When dissolved in groundwater, soluble salts can permeate archaeological materials. They drive reactions of materials in the burial environment, for example the corrosion of iron will be accelerated by availability of chlorides. Where they remain within porous materials, they may cause damage post-excavation by volume changes on crystallisation as humidity falls.
Microorganism activity
The abundance and type of microorganisms within a deposit will depend on a range of factors. Microorganisms that require oxygen and are abundant in moist, well aerated soils. Other types can survive in waterlogged conditions with limited or no oxygen. Microorganisms will not function in completely desiccated environments but these are rare in the UK. Their activity is also related to pH with limited microorganism activity in soils which are strongly acidic or strongly alkaline. The presence of copper or lead can also inhibit microorganism activity. Organic materials are particularly susceptible to biodeterioration by the action of microorganisms.
Physical disturbance
Activities such as ploughing, redeposition, erosion and freeze-thaw action may cause physical damage to archaeological materials in the burial environment. The overburden of soil above the archaeology may also damage finds over time.
Influence of other materials on decay
Mineral preserved organics
Organic materials may be preserved by close proximity to corroding metals in the burial environment. As iron corrodes, mobile corrosion products can permeate adjacent organic materials. The corrosion products prevent the usual decay routes for the organic material and preserve its structure as a pseudomorph (Figure 2.5). Copper ions in solution from corrosion of copper alloy objects can act as a biocide, preventing biological decay of adjacent organic materials. Copper corrosion products may also encase the organic component, preserving its structure.
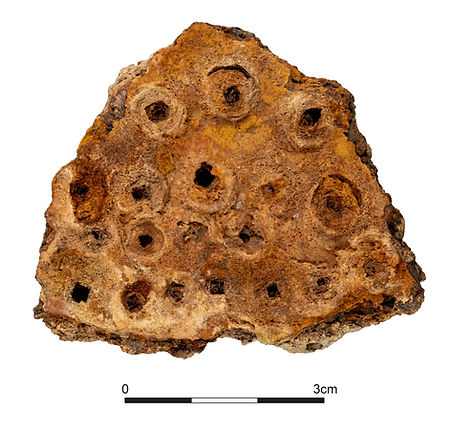

Figure 2.5 Two examples of mineral preserved organics: a leather shoe heel with iron hobnails and a copper alloy ring with organic fibres preserved inside (Images: Cardiff University)
Differential metals
Metals that are in contact with each other in the burial environment are likely to corrode differently. The more reactive metal will corrode in preference to the less reactive metal. This can lead to preferential corrosion of one metal in an alloy or loss of one metal in a composite object of different metals; for example, in a copper alloy with iron fittings, the iron will corrode in preference to the copper alloy components which become protected (Figure 2.6).

Figure 2.6 A buckle showing the preferential corrosion of the iron pin and good condition of the copper alloy (Image: Cardiff University)
Being able to predict which materials might survive in the burial conditions on site can help your planning for first aid. Table 2.4 offers generic guidance for this based on soil pH and water table.

Table 2.4 Possibility of survival of archaeological materials depending on parameters of the burial environment
Further Information
Anon. 2014. Soils as Sponges: How Much Water does Soil Hold?
British Geological Survey (BGS). 2023. Corrosivity Map for the UK.
Cronyn, J. 1990. The Elements of Archaeological Conservation. Routledge, London, UK.
Jordan B. A. 2001. Site Characteristics Impacting the Survival of Historic Waterlogged Wood: A Review. International Biodeterioration & Biodegradation 47(1):47-54.
Neina, D. 2019. The Role of Soil pH in Plant Nutrition and Soil Remediation. Applied and Environmental Soil Science
Price, D. T. and Burton, J. H. 2012. An Introduction to Archaeological Chemistry. Springer, New York, NY.
Reed, S., Bailey, N. and Onokpise, O. 2020. Soil Science for Archaeologists. Florida Agricultural and Mechanical University; Southeast Archaeological Center, National Park Service.
Robinson, W. 1998. First Aid for Underwater Finds. Archetype Publications, London, UK.
Sullivan, C., Thomas, P. and Stuart, B. 2019. An Atomic Force Microscopy Investigation of Plastic Wrapping Materials of Forensic Relevance Buried in Soil Environments. Australian Journal of Forensic Sciences. 51(5): 596-605.
Tokiwa, Y., Calabia, B. P., Ugwu, C. U. and Aiba, S. 2009. Biodegradability of Plastics. International Journal of Molecular Science 10, 3722-3742
Turner-Walker, G. 2008. The chemical and microbial degradation of bone and teeth. In R. Pinhasi and S. Mays (Editors) Advances in Human Palaeopathology. 3-29. Chichester, Wiley.